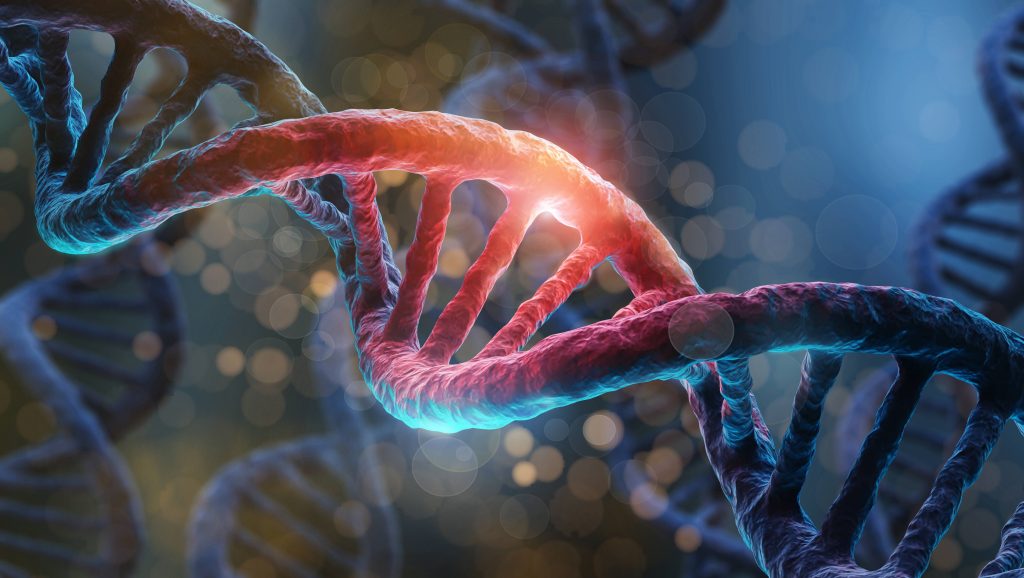

Home » Trends in Biotechnology » CRISPR: The Key to Genome Editing
CRISPR is an innovative technology in the field of biotechnology and genetic engineering, serving as a powerful tool for genome editing. This technology, invented by Jennifer Doudna and her colleague Emmanuelle Charpentier, represents a groundbreaking advancement that holds the potential for treating genetic diseases and revolutionizing the field of medicine.
Contents
How does CRISPR Work?
Bacteria possess an adaptive immune system called CRISPR, which enables them to detect and eliminate viral DNA. One component of this system is the Cas9 protein, which can specifically recognize viral DNA, cleave it and ultimately degrade it. When a cell is infected by a virus, the viral DNA is injected into the cell. In bacteria, the CRISPR system allows the viral DNA to be copied and incorporated into a specific location called the CRISPR locus as short palindromic repeat sequences known as “spacers”. Portions of the viral DNA are then passed on to subsequent generations of cells, effectively creating a genetic vaccination card for the cells.
How was The CRISPR Technology Developed?
CRISPR originally emerged as a system that provided researchers with the ability to make significant and precise changes to DNA sequences. It started as a fundamental research project aimed at understanding how bacteria combat viral infections. Doudna and her team discovered that Cas9 could be used as a genetic engineering tool to edit DNA in cells, opening up unprecedented opportunities for removing or inserting specific DNA sequences that were previously inaccessible. Since then, CRISPR has been employed for targeted and precise DNA modifications in various organisms, including mice, monkeys, and even human embryos.
CRISPR-Cas9 Mechanism of Action
The CRISPR system consists of the Cas9 protein and a molecule called RNA, which precisely matches a viral DNA code. RNA binds to the Cas9 protein, forming a complex that functions as a sentinel within the cell. This complex searches through the entire cell’s DNA to find locations that match the sequences present in the RNA. When a match is found, the Cas9 protein creates a precise cleavage in the viral DNA, leading to its destruction. This complex can be programmed to recognize and cleave specific DNA sequences, allowing scientists to identify and eliminate targeted DNA segments. This process is somewhat akin to an automated text editor. The efficiency of CRISPR for genome engineering lies in the cells’ ability to recognize and repair broken DNA. When a cell detects a double-strand DNA break, it can repair the break by either rejoining the broken DNA ends with a small sequence alteration or inserting a new piece of DNA. This repair mechanism empowers cells to create precise changes in DNA at the site of the break, providing a controlled and precise approach to gene editing. CRISPR requires a DNA carrier molecule to guide the repair process, and this is where the CRISPR technology comes into play. By introducing a break in the DNA helix using Cas9, we can activate the cell’s repair mechanism to delete specific genes, insert new genes, or create targeted mutations, resulting in highly precise changes to the DNA sequence. This opens up new frontiers of genetic engineering for various organisms, including humans.
Examples of CRISPR Applications
In the field of medicine, CRISPR has revolutionized the editing of human cell DNA, offering potential treatments for genetic diseases like sickle cell anemia and genetic blindness. In agriculture, CRISPR holds immense promise in developing disease-resistant crops, plants resistant to pests, and enhancing adaptability to diverse environmental conditions, thereby addressing global food security challenges. Additionally, CRISPR has also found applications in environmental conservation, where it can be used to edit the DNA of endangered species, thereby increasing their chances of survival.
What sets CRISPR apart from older technologies and makes it invaluable is its relative simplicity compared to other methods. Unlike previous approaches that were often inefficient or challenging, CRISPR can be easily programmed using small RNA molecules. This remarkable feature opens up promising avenues for correcting mutations that lead to genetic disorders such as sickle cell anemia and Huntington’s disease.
To study the precise effects of DNA alterations on tissues and organisms, researchers employ animal models such as mice and monkeys. For instance, CRISPR has been used to disable a gene responsible for skin color in mice, resulting in a partial DNA modification that turns their fur white while preserving other natural characteristics.
Overall, CRISPR represents a revolutionary technology with the potential to transform various aspects of biotechnology, medicine, agriculture, and more. However, it also raises significant ethical and societal concerns that require attention. By adopting a cautious and responsible approach to the development and use of CRISPR, we can harness its power for the benefit of humanity while ensuring its applications align with ethical principles and serve the best interests of society.